Creating Circularity in the Phosphor and Lighting Waste Supply Chain
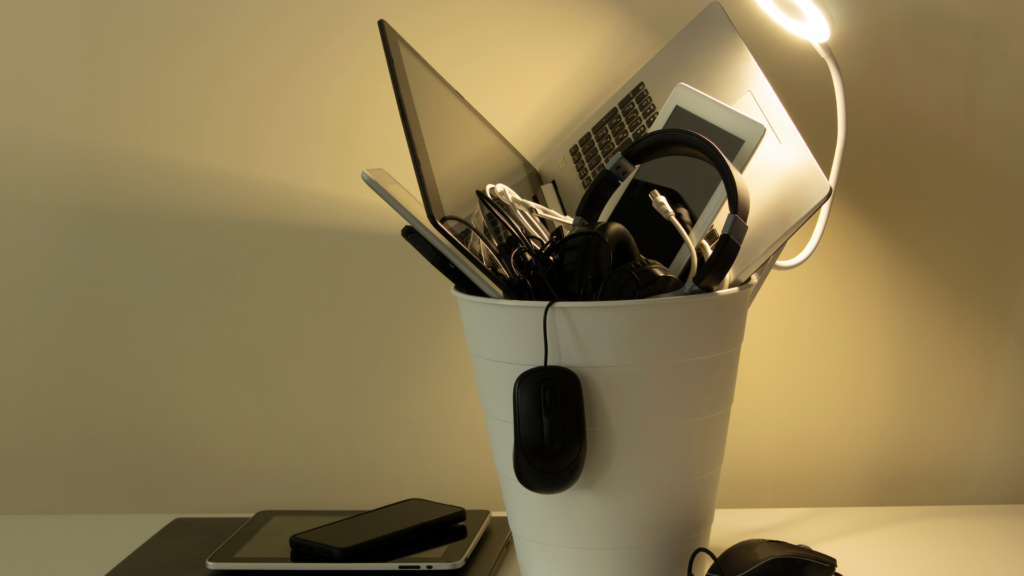
Welcome to the enlightening world of phosphor and lighting waste circularity! On this thrilling journey, we’ll delve into the escalating demand for Rare Earth Elements (REEs) and the challenges posed by mining. Living in a globe bathed in artificial light, have you ever wondered about the waste generated and REEs lost? Alarmingly, it’s a bigger issue than we realize. But fret not – the good news is we’re proposing a solution: circularity! If we manage to extract Yttrium, Europium, and Terbium efficiently from waste, we’re on the way to creating a sustainable recycling loop. Ready to explore more? Let’s step into the ‘circular’ realm of science, pre-treatment, extraction methods, and the wonders of reintroducing these elements into new products. Perks? Energy conservation, hazardous mitigation, and even job creation! Let’s illuminate the pathway to a sustainable, circular economy, shall we?
Phosphor Science and Its Challenges
Among key concerns in the lighting waste supply chain are the implications surrounding phosphor science. An essential aspect to understand is the composition of phosphors in lighting, which is largely comprised of CFL and LED phosphors and has a significant role of Rare Earth Elements (REE).
Composition of Phosphors in Lighting
CFL and LED Phosphors
The use of phosphors varies significantly when comparing Compact Fluorescent Lights (CFLs) and Light Emitting Diodes (LEDs). CFLs exhaust phosphors to produce white light, which can become a concern when these bulbs reach their end of life and become waste. On the other hand, LEDs use phosphors to convert high-energy blue light to a full spectrum white light, leading to different phosphor compositions and recycling challenges.
Role of Rare Earth Elements (REE)
Critical to phosphor science is the role of REEs, which are crucial for enabling the bright and vibrant colours we see in modern display technologies. REEs such as Yttrium (Y), Europium (Eu), and Terbium (Tb) serve significant functions in phosphors, making their recovery and reuse of strategic importance given their supply risk and environmental implications.
Challenges in Phosphor Recycling
Mixtures and Contaminants
One prominent hurdle in phosphor recycling pertains to the handling of mixtures and contaminants. The presence of different phosphors types and other materials in the waste can complicate the recycling process, often leading to reduced purity of recovered elements.
Phosphor Degradation
A further complication arises from phosphor degradation. Over time and usage, the properties of phosphors change, affecting their quality and thus the viability of their reuse. Future innovative recycling processes must address these challenges and find convenient solutions for recovering elements of interest from degraded phosphors, all while ensuring minimal environmental impact.
Through better appreciating these phosphor science challenges, efforts can be steered towards crafty solutions for a robust, circular phosphor and lighting waste supply chain.
Collection and Pre-Treatment of Lighting Waste
The initial step in creating a circular supply chain for phosphor and lighting waste involves careful classification, collection, and pre-treatment.
Classification of Lighting Waste
Among the myriad of electrical waste, a categorical distinction is crucial for efficient handling. For our discussion, two main classifications stand out: Lamps and LEDs.
Lamps versus LEDs
Lamps, primarily CFLs (compact fluorescent lamps), contain phosphors and mercury, a toxic element that poses handling and disposal challenges. LEDs (light emitting diodes), on the other hand, are mercury-free but still contain valuable REEs (Rare Earth Elements) within their phosphor coatings, which warrant serious recycling consideration.
Infrastructure for Waste Collection
Successful circularity also requires a robust and sustainable collection system. This is realized through two primary mechanisms: Municipal and Industrial Systems and Take-Back Programs.
Municipal and Industrial Systems
Municipal waste management systems form the backbone of traditional waste collection. However, including industrial partners can enhance the scope and efficiency of collection, particularly for large waste producers.
Take-Back Programs
Increasingly, manufacturers and retailers are implementing take-back programs, encouraging consumers to return used lighting products to designated collection centers. This not only helps to conveniently segregate lighting waste but also enhances recycling rates.
Pre-Treatment Processes
Before extraction methods can be employed, pre-treatment processes are necessary for the removal of hazardous materials and the mechanical separation of components.
Hazardous Material Removal
Used lamps can contain harmful substances, such as mercury, necessitating careful removal and safe disposal. Mercury abatement technologies and protocols are employed to mitigate this health risk.
Mechanical Separation Techniques
Following hazardous material removal, mechanical processes are used to separate the lamp components: the glass, metal end caps, phosphor powder, and, in the case of fluorescent lamps, the mercury-bearing phosphor powder.
As these stages illustrate, the systematic collection and pre-treatment of lighting waste are critical steps toward a circular supply chain, turning lighting waste into a valuable resource rather than an environmental burden. Future improvements in these areas will hinge on innovations in technology and policy alike.
Extraction Methods for REE from Lighting Waste
Redirecting our focus onto the heart of the phosphor waste problem – recovering the vital rare earth elements (REE) – it becomes clear that we have an array of methods available. These can broadly be classified into traditional hydrometallurgical techniques and more advanced, environmentally conscious methods that have been emerging recently.
Hydrometallurgical Techniques
Hydrometallurgy introduces chemical solutions to extract precious materials; it primarily employs two primary techniques for waste processing: acid leaching and solvent extraction.
Acid Leaching Processes
In acid leaching, an acidic solution is used to dissolve the REE, allowing for their subsequent separation. While this method is highly effective, the use of harsh acids raises environmental concerns, as uncontrolled acid leakage can lead to soil and water pollution.
Solvent Extraction Approaches
Solvent extraction provides another alternative. It involves using organic solvents to selectively extract REE from the leaching solution. Particular care must be taken with handling and disposal of these solvents given their often toxic nature.
Advanced and Green Extraction Methods
On the other hand, the pursuit of green and sustainable practices has ushered in a range of unconventional extraction methods.
Bioleaching
Bioleaching enlists microorganisms to do the hard work. Certain bacteria are capable of dissolving REE from waste materials, offering an environmentally friendly and cost-effective alternative to traditional acid leaching.
Use of Ionic Liquids
Ionic liquids — salts in a liquid state— are adjustable and less harmful to the environment, making them an appealing option for REE extraction.
Electrochemical Methods
Enter electrochemical methods, which leverage electric currents to extract REE. These methods stand out for their high efficiency and specificity, as well as their low environmental impact.
Supercritical Fluid Extraction
Finally, a special mention goes to supercritical fluid extraction, which uses substances at critical temperature and pressure as extraction media. These conditions remove the boundary between liquid and gas phases, with sub-critical water showing promise as an eco-friendly medium for REE extraction.
Comparative Analysis of Extraction Techniques
When it comes to weighing up these extraction methods, a few key factors stand out.
Efficiency and Cost Considerations
Naturally, the efficacy and finance implications of the techniques largely dictate their usability. Acid leaching and solvent extraction, despite their environmental challenges, remain popular due to their high efficiency and relatively low costs. In contrast, while eco-friendly methods like bioleaching and ionic liquids promise sustainability, they often fall behind in efficiency and/or come with high operational costs.
Environmental Impacts
The environmental impact of each method should be a primary consideration. We must balance the immediate benefits of high-efficiency traditional approaches with the longer-term sustainability of greener methods. Moreover, the circular economy approach to phosphor and lighting waste must ensure minimal environmental harm during the extraction phase.
In conclusion, a multi-pronged approach that utilizes the strengths of both conventional and advanced methods may well be the key to making phosphor and lighting waste circular – a field that is ripe for research and exploration.
Closing the Loop in Lighting Waste Circularity
In the endeavor to promote circularity in the phosphor and lighting waste sector, it’s crucial that we focus on “Closing the Loop.” This phase involves redirecting recycled rare-earth elements (REE) back into the manufacturing cycle – a process that features two key components: reintegrating recycled materials and maneuvering policy implications.
Reintegrating Recycled Materials
The first facet of closing the loop concerns integrating the extracted REEs into the production of fresh materials, notably in the production of new phosphors and LEDs. Incorporating recycled REEs into the production of new compact fluorescent lamps (CFLs) and light-emitting diodes (LEDs) not only lessens the demand for freshly mined resources, but also mitigates the environment degradation linked to REE mining.
Another avenue for the application of these recycled materials lies in the crafting of magnets and batteries. Being key constituents of numerous technologies, ranging from electric vehicles to renewable energy systems, the need for REEs is on a sharp ascent. By channeling the recycled REEs into these sectors, we foster circularity and push back against the linear process of use and discard.
Economic and Policy Considerations
The pathway to a circular REE economy doesn’t solely rely on technological innovation; it’s a dance with policy, economics, and technology. A meaningful shift requires a delicate blend of incentives and extended producer responsibility (EPR). EPR policies urge manufacturers to take accountability for the entire life cycle of their products, guiding them to create more sustainable products. Simultaneously, fiscal incentives can stimulate the recycling sector, encourage innovation, and prop up end markets for recycled materials.
Finally, global trade implications come into play, stirring up the dynamics of REE circulation. Trade regulations and tariffs, combined with geopolitical tensions, can dictate the feasibility of both REE mining and recycling on a global scale. Therefore, a robust and resilient recycled REE market must factor in these complexities to create a truly circular economy.
By intertwining these intricate strands – technical, economic, and policy – we can realistically envision and construct a circular supply chain for phosphor and lighting waste, marking a giant leap towards material sustainability and resource conservation.
Environmental and Social Impacts of Circular Lighting Waste Management
With the rise in the adoption of circular patterns in lighting waste management, there are significant environmental and societal impacts. A well-managed cycle creates opportunities to reduce environmental damage while offering substantial societal benefits.
Reducing Environmental Impact
Utilizing processes aiming at circularity in lighting waste can make an unparalleled contribution to reducing environmental degradation. Lighting waste, when managed linearly, holds vast potential for environmental harm due to the substantial energy requirement and massive carbon footprints accompanying the manufacturing of fresh components.
Energy and Carbon Footprint
Recycling processes embedded in a circular system reduce energy consumption as they eliminate the need to extract and refine raw materials. This reduction leads directly to minimized carbon footprints, serving to combat the overbearing environmental concern that is climate change.
Mitigating Hazardous Waste
Lighting components, notably Compact Fluorescent Lamps (CFLs) and Light Emitting Diodes (LEDs), contain a broad host of hazardous elements like mercury. Circular waste management methodologies give importance to the safe and efficient extraction and recycling of these substances, thereby mitigating risks of these toxic materials infiltrating the environment.
Societal Benefits
Adjacent to the environmental benefits are societal gains that are integral to the adoption of circular waste management strategies. At the heart is the creation of jobs and, subsequently, opportunities for economic development.
Job Creation in Urban Mining
Urban mining, the process of recovering raw materials from waste, can exhibit employment opportunities in areas such as collection, sorting, and upcycling operations. Thus, by pushing lighting waste into well-defined circular systems, urban mining occupations can increase significantly.
Community Health Improvements
Furthermore, circular waste management strategies prioritize the safe handling and processing of hazardous substances, having a direct impact on improving local communities’ health. By safeguarding against potential toxic contamination of the environment, the quality of life improves, underlining the enormity of societal benefits accompanying circular waste management.
As we delve further into the details of phosphor and lighting waste circularity, the environmental and social impacts become increasingly more prominent. Balancing these advantages while overcoming challenges is a test for future development in this field. But with continued collaboration and research, a circular system that benefits all beings might just be within our reach.
Overcoming Challenges and Future Prospects
Progressing towards a circular economy in phosphors and lighting waste supply chain teems with challenges. While the process is daunting, it’s also filled with opportunities for innovation and problem-solving solutions as we pivot toward sustainability.
Technical and Logistical Challenges
Separation and Collection
The first hurdle is the separation and collection of used lighting products. Current collection methods largely depend on consumers’ willingness and awareness to recycle. Moreover, separating the phosphors, a key component in the lights, from the rest of the waste is a significant challenge due to the minute and dispersed nature of these elements. Innovative approaches to the collection mechanism, perhaps via smart bins or dedicated recycling centers, may warrant exploration.
Purity of Extracted Materials
Another concern lies with the purity of extracted materials, imperative for their reusability. The extraction process needs to ensure minimal contamination, which requires sophisticated technologies and rigorous monitoring.
Market and Economic Challenges
Competition and Price Volatility
In terms of market challenges, the foremost issue is competition and price volatility. Freshly mined resources are still cheaper than recycled counterparts due to their high quality and quantity. Lighting waste recycling, especially for rare earth elements (REE), is expensive. In addition, REE prices are notoriously volatile, making investment decision-making a risky business. Therefore, advocating for economic policies that favor recycling exploration like tax incentives or grants will be pivotal.
Research and Development Roadmap
Emerging Technologies
Looking beyond the immediate hurdles, though, there are plenty of opportunities for progress. One exciting field encompasses the growth of emerging technologies such as advanced extraction techniques and artificial intelligence (AI) in managing waste segregation. These innovations allow for higher efficiency while also reducing environmental impact.
Collaboration Opportunities
Another promising avenue involves seeking collaboration opportunities. Companies, governments, and academia must work together to increase R&D for recycling technologies, draft stringent yet supportive policies, and, importantly, spread awareness about the importance and benefits of circular approaches to lighting waste management.
All said and done, overcoming these challenges and prospecting for future advancements can pave the way for a sustainable phosphor industry that aligns with the ideals of a circular economy.
Policy Development and Partnerships
In the quest to establish circularity in phosphor and lighting waste supply chain, policy development and partnerships play pivotal roles.
Regulatory Frameworks
Foremost in regulatory frameworks are the Extended Producer Responsibility (EPR) Schemes.
Extended Producer Responsibility (EPR) Schemes
EPR schemes are regulatory measures intended to promote the integration of environmental costs associated with goods throughout their life spans into the market price of the products. In the context of phosphor and lighting waste, producers, under EPR schemes, bear significant responsibility for the treatment or disposal of post-consumer products. This shift of responsibility, coupled with the adoption of appropriate recycling technologies, could incentivize producers to design environmentally-friendly products and provide efficient recovery and recycling services. EPR schemes, when enforced, have the potential to drastically reduce the inefficiencies and losses in the phosphor and lighting waste supply chain.
Incentivizing Circular Systems
In addition to regulations, government incentives are paramount in fostering circularity in phosphor and lighting waste.
Government Incentives
Facilitative policies, tax reductions or exemptions for eco-designed products/ processes and financial support for research and development of recycling technologies, such as supercritical fluid extraction, bioleaching, and electrochemical methods, can bring about a paradigm shift. The key is to incentivize not just the recycling and recovery of rare-earth elements from waste but also the reuse of these recovered elements in the production of new phosphors, LEDs and other applications. These incentives can spur innovation and foster cooperation among various stakeholders in the supply chain, paving the path towards a more circular economy in phosphor and lighting waste management.
Conclusion and Future Directions
Summary of Key Findings
As we conclude this journey into the world of lighting waste circularity, key findings have emerged. The complexity of the phosphor composition, the challenges in collection and pre-treatment processes, and extraction methodologies have provided in-depth understanding of the lighting waste problem. Innovative solutions, including hydrometallurgical techniques and advanced, green extraction methods, have been explored to make this process more efficient and sustainable.
Urgency and Next Steps
This insight into lighting waste circularity isn’t merely academic. With growing demand for Rare Earth Elements (REE), and considering the environmental impact of mining, there is a palpable sense of urgency. The next steps demand orchestrated efforts towards research in emerging technologies, policy reformation and the development of an effective global trade mechanism.
Potential of Circular Economy Solutions
The potential of circular economy solutions in this context is vast. By closing the loop, not only can we alleviate pressure on mining but also trigger a wave of job creation in urban mining. Moreover, carefully planned recycling strategies and reintegration of recycled materials into the economy can drastically reduce our carbon footprint, providing an impetus for more sustainable future.
Importance of Collaboration
Finally, this endeavour underscores the importance of collaboration among industries, policymakers, researchers and society at large. As we move forward, partnerships will bridge gaps between policy and practice, and steer us towards alignment between technological advancements and market needs. The scale of these challenges calls for a collective response, making collaboration not merely beneficial, but indispensable.